文章信息
- 常泽, 王立成
- CHANG Ze, WANG Licheng
- 混凝土内部温度场的细观数值模拟研究
- Mesoscopic numerical simulation of the temperature field of concrete
- 武汉大学学报(工学版), 2017, 50(6): 938-942
- Engineering Journal of Wuhan University, 2017, 50(6): 938-942
- http://dx.doi.org/10.14188/j.1671-8844.2017-06-021
-
文章历史
- 收稿日期: 2017-06-13
实际环境中的混凝土结构,往往承受着温度、湿度及应力等多场的耦合作用.混凝土内部温度分布不均匀产生的梯度,是引起混凝土开裂、影响其使用寿命的一个重要因素[1-3].近些年来,许多学者开展了关于温度场对混凝土开裂影响的机理分析,但大多数研究主要集中在关于导热系数方面[4-6].Zhang等[7]综合考虑了饱和度、材料导热系数、骨料含量等因素,建立了非饱和状态下混凝土热传导系数的细观模型;Demirboĝa[8]研究了混凝土内部的矿物掺和料对混凝土导热系数的影响,对比分析了不同掺和料的作用效果;唐世斌[9]通过对混凝土热传导与热应力细观特性的分析,开展了细观非均匀性对混凝土热学和力学性能影响的研究.本文利用Voronoi单元划分建立细观格构网络模型,以组成混凝土的水泥砂浆与花岗岩为例,利用一维的非线性热传导方程,开展基于细观格构网络模型的热传导过程数值分析.
1 细观格构网络模型根据材料的组成和内部结构特点,通常将混凝土研究分为宏观、细观和微观3个层次.在宏观层次上,将混凝土视为均质、各向同性材料,宏观层次的划分难以体现混凝土内部粗骨料与水泥砂浆热传导性能的差异,无法反映混凝土内部温度场的不均匀分布;在微观层次上,材料的结构尺寸在分子、原子量级,将混凝土视为由砂浆基质和孔隙组成的非均质材料;在细观层次上,混凝土通常被视为由水泥砂浆、骨料以及二者界面过渡区(ITZ)组成的三相复合材料(图 1),将连续介质离散为由弹性杆或梁单元连结而成的格构网络系统.目前来看,已有学者基于细观格构网络模型开展了有关混凝土及其他非均质材料物质传输[10, 11]和力学性能[12, 13]的相关研究.
![]() |
图 1 混凝土细观结构模型 Figure 1 Mesoscale structure model for concrete |
本文基于细观层次,利用混凝土细观结构模型, 按照Voronoi图形原理进行单元划分,得到由凸多边形随机生成的几何单元图,将Voronoi单元的中点与各边的中点直线连结,形成Voronoi单元内部的格构单元,其中Voronoi单元的边界均形成2个边界格构单元.根据这一划分原则,形成砂浆格构单元、骨料格构单元、砂浆与砂浆界面格构单元、骨料与骨料界面格构单元以及砂浆和骨料界面格构单元组成的混凝土的细观格构网络模型,如图 2所示.
![]() |
图 2 格构网络模型示意图 Figure 2 Schematic diagram of lattice network model |
由上述分析可知,基于该模型,混凝土在二维平面内的热传导问题可由一维格构单元组成的网络来代替,根据其格构单元的类型确定不同格构单元的热传导系数.Voronoi单元内部的格构单元,截面面积取对应三角形mnj面积,热传导系数根据其格构单元类型取值;对于边界格构单元,当考虑开裂时,根据裂缝的位置以及宽度决定单元的截面面积与热传导系数.
2 热传导方程及数值方法混凝土内部温度场的分布主要是受外界环境温度以及自身因素的影响[14-16],基于傅里叶定律,可知混凝土的热传导方程为
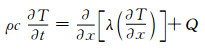
式中:T为绝对温度;ρ为材料的质量密度;c为材料的比热容;λ为材料的有效导热系数,主要与粗骨料含量、湿度条件有关;Q为热源,主要针对早龄期混凝土的水泥水化放热和水分蒸发散热形成,因此,对于处于正常工作条件下的混凝土,水化热可以忽略不计,即Q=0.
若混凝土导热系数λ为常数,考虑混凝土的初始条件与边界条件,可以推导出解析解为

式中:T0为某点处的初始温度;Ts为模型边界的温度;x为距离边界的距离;Dt=λ/(ρc).
当热传导系数随时间变化时,式(1)具有高度的非线性,利用伽略金加权余数法和有限差分法确定方程的数值形式.对于长度为L的一维格构单元ij,其加权余函数可定义为
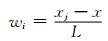
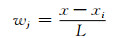
温度的任意函数F(T)沿格构直线单元ij的加权余函数可表示为

于是,根据伽略金加权余数的原则可知:
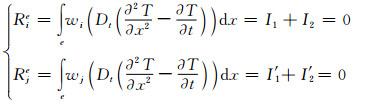
利用分部积分法分别计算I1、I2:
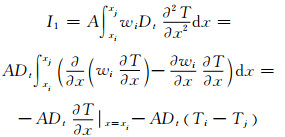
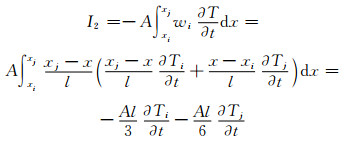
同理可求

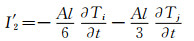
最后整理可确定单个格构单元ij的有限元方程形式为
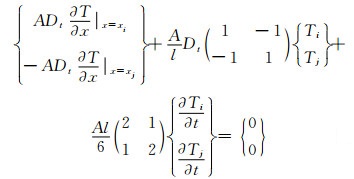
其简化形式为
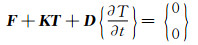
式中:
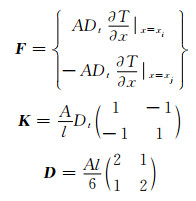
式中:A为单个格构单元的面积; L为格构单元的长度.
采用时间步长Δt的Crank-Nicholson差分法:


综上所述,可知一维热传导方程的数值形式为
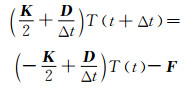
本文以水泥砂浆与花岗岩为例进行分析计算,探讨混凝土的各组成成分对于内部温度场的影响,将数值计算结果与理论解析解对比,验证所建立的格构网络模型的适用性.
试样的暴露面温度为50 ℃,其他3面温度为20 ℃,试件的初始温度为20 ℃.建立如图 3所示的细观格构网络模型,材料参数如表 1所示.
![]() |
图 3 水泥砂浆(花岗岩)热传导计算模型 Figure 3 Calculation model of thermal conduction in mortar (granite) |
材料类型 | 导热系数λ/(W·m-1K-1) | 密度ρ/(kg·m-3) | 比热容c/(J·kg-1℃-1) | 热扩散率Dt/(mm2·min-1) |
水泥砂浆 | 1 | 1 800 | 1 050 | 31.74 |
花岗岩 | 3.1 | 2 800 | 920 | 72 |
基于细观格构网络模型,计算硬化水泥砂浆与花岗岩材料在时间为5、10、15 min的热传导情况(图 4、5),并得到了对应的温度分布云图(图 6、7),同时,比较分析传导时间为10 min时水泥砂浆与花岗岩温度数值,如图 8所示.
![]() |
图 4 不同时间的水泥砂浆热传导 Figure 4 Temperature profiles of mortar after different times |
![]() |
图 5 不同时间的花岗岩粗骨料热传导 Figure 5 Temperature profiles of granite after different times |
![]() |
图 6 水泥砂浆温度场分布云图 Figure 6 Thermal distribution diagram of mortar |
![]() |
图 7 花岗岩温度场分布云图 Figure 7 Thermal distribution diagram of granite |
![]() |
图 8 水泥砂浆与花岗岩热扩散对比 Figure 8 Comparison of thermal conduction profiles between mortar and granite |
基于细观格构网络模型的热传导分析,考虑了混凝土的非均质性,通过数值计算得到不同时间下的水泥砂浆与混凝土粗骨料花岗岩的温度数值解与分布云图.从图 4、5可知,混凝土中花岗岩与水泥砂浆温度场数值模拟值与理论计算值吻合较好,说明采用细观格构网络模型将混凝土二维的热传导问题进行一维的简化是准确的,该数值计算方法能够对混凝土内部的温度场分布做出精确的计算.对比图 6、7可以看出,由于花岗岩的热传导性能比水泥砂浆的热传导性能好,因此相同时间下,骨料温度场分布云图的变化大于水泥砂浆.分析图 8可以看出,相同传导时间下,水泥砂浆温度场变化较大,主要原因是相比水泥砂浆,花岗岩的热扩散率更大.
综上所述,将水泥砂浆与粗骨料区别对待的细观格构网络模型可以用于计算混凝土温度场的分布,该方法利用一维格构单元组成的网络解决了二维平面内的热传导问题;同时,细观格构网络模型下分析混凝土的热传导性能,将水泥砂浆与花岗岩区划分为不同单元,可以有效地模拟混凝土温度场的分布.
4 结论实际环境的混凝土结构,有关温度传导特性的研究已经越来越受到重视.本文首先从细观层次分析了混凝土的结构组成,基于细观格构网络模型对于混凝土的温度场分布进行计算求解.
细观格构网络模型是利用一维格构单元组成的网络解决了二维平面内的热传导问题,这种方式考虑了混凝土的非均质性,砂浆、粗骨料、界面单元按照不同的类型选取热传导系数,借助细观网络格构模型可以准确地预测混凝土内部温度场的分布,为分析混凝土的力学性能提供依据.
[1] |
戴荟郦. 周期性温湿度作用下混凝土内部温湿度场的模拟计算[D]. 合肥: 安徽工业大学, 2014.
Dai Huili. Simulation of temperature and humidity field in concrete under cyclical temperature and humidity[D]. Hefei: Anhui University of Technology, 2014. |
[2] |
吴蒙. 水工混凝土湿热耦合应力研究[D]. 北京: 清华大学, 2008.
Wu Meng. Stress analysis of hydraulic concrete based on coupled heat-moisture transfer[D]. Beijing: Tsinghua University, 2008. |
[3] |
陈德鹏. 基于多物理场耦合的混凝土湿热变形数值模拟[J].
东南大学学报(自然科学版), 2013, 43(3): 582–587.
Chen Depeng. Multi-physical field coupling simulation of hygro-thermal deformation of concrete[J]. Journal of Southeast University (Natural Science Edition), 2013, 43(3): 582–587. DOI:10.3969/j.issn.1001-0505.2013.03.025 |
[4] |
肖建庄, 宋志文, 张枫. 混凝土导热系数试验与分析[J].
建筑材料学报, 2010, 13(1): 17–21.
Xiao Jianzhuang, Song Zhiwen, Zhang Feng. An experimental study on thermal conductivity of concrete[J]. Journal of Building Materials, 2010, 13(1): 17–21. |
[5] |
张伟平, 童菲, 邢益善, 等. 混凝土导热系数的试验研究与预测模型[J].
建筑材料学报, 2015, 18(2): 183–189.
Zhang Weiping, Tong Fei, Xing Yishan, et al. Experimental study and prediction model of thermal conductivity of concrete[J]. Journal of Building Materials, 2015, 18(2): 183–189. |
[6] |
任青文, 沈雷, 孙立国, 等. 开裂混凝土有效导热系数的细观数值研究[J].
水利学报, 2015, 46(8): 892–899.
Ren Qingwen, Shen Lei, Sun Liguo, et al. Numerical study of the influence of cracks on effective thermal conductivity of concrete in meso-scale[J]. Journal of Hydraulic Engineering, 2015, 46(8): 892–899. |
[7] | Zhang W, Min H, Gu X, et al. Mesoscale model for thermal conductivity of concrete[J]. Construction and Building Materials, 2015, 98: 8–16. DOI:10.1016/j.conbuildmat.2015.08.106 |
[8] | Demirboĝa R. Thermal conductivity and compressive strength of concrete incorporation with mineral admixtures[J]. Building and Environment, 2007, 42(7): 2467–2471. DOI:10.1016/j.buildenv.2006.06.010 |
[9] |
唐世斌. 混凝土温湿型裂缝开裂过程细观数值模型研究[D]. 大连: 大连理工大学, 2009.
Tang Shibin. Mesoscopic numerical model of thermo-hygro cracking process in concrete[D]. Dalian: Dalian University of Technology, 2009. |
[10] | Wang L, Soda M, Ueda T. Simulation of chloride diffusivity for cracked concrete based on RBSM and truss network model[J]. Journal of Advanced Concrete Technology, 2008, 6(1): 143–155. DOI:10.3151/jact.6.143 |
[11] |
鲍玖文. 混凝土中物质传输过程的细观数值模拟研究[D]. 大连: 大连理工大学, 2014.
Bao Jiuwen. Numerical simulation on mass transport process in concrete by the mesoscale method[D]. Dalian: Dalian University of Technology, 2014. |
[12] |
金浏. 细观混凝土分析模型与方法研究[D]. 北京: 北京工业大学, 2014.
Jin Liu. Study on meso-scopic model and analysis method of concrete[D]. Beijing: Beijing University of Technology, 2014 |
[13] |
汤书军. 混凝土材料细观力学模型与破坏分析[D]. 南京: 河海大学, 2006.
Tang Shujun. Mesomechanical model and failure analysis of concrete material[D]. Nanjing: Hohai University, 2006. |
[14] |
唐世斌, 唐春安, 梁正召, 等. 混凝土热传导与热应力的细观特性及热开裂过程研究[J].
土木工程学报, 2012, 45(2): 11–19.
Tang Shibin, Tang Chunan, Liang Zhengzhao, et al. Study of thermal conduction and thermal stress of concrete at mesoscopic level and its thermal cracking processes[J]. China Civil Engineering Journal, 2012, 45(2): 11–19. |
[15] | Chen D, Mahadevan S. Cracking analysis of plain concrete under coupled heat transfer and moisture transport processes[J]. Journal of Structural Engineering, 2007, 133(3): 400–410. DOI:10.1061/(ASCE)0733-9445(2007)133:3(400) |
[16] | Isgor O B, Razaqpur A G. Finite element modeling of coupled heat transfer, moisture transport and carbonation processes in concrete structures[J]. Cement and Concrete Composites, 2004, 26(1): 57–73. DOI:10.1016/S0958-9465(02)00125-7 |
[17] | Kim K H, Jeon S E, Kim J K, et al. An experimental study on thermal conductivity of concrete[J]. Cement and Concrete Research, 2003, 33(3): 363–371. DOI:10.1016/S0008-8846(02)00965-1 |